Few things in life are more important than the food we consume. Today, our food supply is more diverse and more highly processed than ever before. To ensure the safety and nutritional quality of our food many countries have promulgated regulations that stipulate acceptable levels for individual chemical additives, residues and contaminants in food products. Other regulations require food packaging to list ingredients relating to nutritional content, such as unsaturated and saturated fat. Food manufacturers and processors themselves must be able to assess product quality. Meeting all of these requirements is the function of food analysis. Table 1 provides an overview of the major classes and subclasses of food analytes and includes typical examples and function.
Increasingly, food analysis methods are built around high-performance liquid chromatography (HPLC), which has proven to be an optimal technology for detecting and/or quantifying the vast majority of food analytes. These methods employ a stepwise approach that first removes the sample matrix, then isolates the analytes of interest and individually resolves them on a chromatographic column. The efficiency of the separation depends on, among other things, the differential interaction of analytes of interest with both mobile and column stationary phases. Of course, classifying food analytes according to their relative volatility and polarity are factors that must be considered when selecting an appropriate analytical method for their determination.
SAMPLE PREPARATION
Typically, the first stage of HPLC analysis is sample preparation, which involves the introduction of a medium into which the target analytes can be preferentially partitioned followed by the separation of the analyte-containing phase from the remainder of the sample. The selection of the partitioning medium is determined by the nature of the sample matrix and the properties of the analytes. In addition, the sample may be heated, have its pH adjusted, or be otherwise treated to make the analytes more accessible to the partitioning medium.
Solids. Samples must be representative of the material being assayed, and if not uniform, are homogenized prior to analyte partitioning. There are three types of extraction readily used for solid food analytes. Liquid extraction enables highly selective matching of solvent to analyte solubility. Extraction times are short; typically minutes. This technique uses relatively small quantities of organic solvents, thereby reducing costs and facilitating disposal. Ultrasonic stimulation is often applied in conjunction with the extraction solvent to drive the liquid medium into the interior of the matrix, ensuring intimate and energetic contact between solvent and analyte molecules. When analytes are thermally stable, solvents may be heated to increase their solvating power or to accelerate matrix penetration. Samples containing thermally sensitive analytes may require active cooling to prevent degradation.
Supercritical fluids have properties intermediate between a gas and a liquid, facilitating penetration of the sample matrix. Carbon dioxide is the preferred supercritical fluid as it is readily available, nontoxic, nonpolluting, and becomes supercritical at relatively low pressures and temperatures. Dissolved analytes are easily recovered by releasing the pressure, thereby returning CO2 to the gaseous state. CO2’s low polarity limits its solvating power, but this can be remedied somewhat by the addition of small amounts of polar solvents such as methanol. As with liquid extraction, supercritical extraction times are short and the method is amenable to automation. However, ultrapure supercritical fluids for trace analysis are not always available.
Steam distillation can be utilized to selectively extract volatile compounds. Extraction times are long, however, and the method has a narrow range of use and is applied offline.
Irrespective of the extraction process, analytes ultimately must be placed in solution for chromatographic separation. This solution may be subjected to additional pretreatment, such as liquid/liquid extraction (LLE) or solid-phase extraction (SPE), to remove substances that might interfere with the chromatographic separation and the detection of the target analytes.
Liquids. Liquid-liquid extraction, on- and offline solid-phase extraction and gel permeation chromatography (GPC) are used in the partitioning of target analytes in liquid samples or liquid extracts of solid samples. Turbid or otherwise opaque liquids are usually filtered to remove particulates prior to extraction.
LLE enables the selection of a solvent system tailored to maximize partitioning of the analytes of interest. Extractions can be carried out manually with separatory funnels or in automated fashion using continuous or counter-current distribution apparatus. The method is both simple and versatile as it can be combined with highly selective modifiers (pH, salts, or ion-pairing reagents). Drawbacks include the need for large amounts of potentially toxic solvents that may present potential health hazards and/or environmental disposal problems.
Solid-phase extraction typically is used to capture target analytes in adsorbent filled cartridges from which they are recovered by elution with an appropriate solvent. A range of available materials provides a choice of selectivities, enabling SPE to be used both for concentrating and fractionating. SPE is one of the fastest-growing sample preparation and cleanup techniques and efforts are ongoing to automate the procedure and integrate it with chromatography using systems that employ both robotics and programmable valves.
Gel permeation chromatography, also known as size-exclusion chromatography (SEC), has become a standard technique for separating high and low molecular weight compounds. In GPC, compounds are eluted in order of decreasing molecular weight (size).
CHROMATOGRAPHY
When performing HPLC, guard columns often are installed at the head of the analytical column to prevent contamination of the stationary phase by the sample matrix. The guard column connected to the precolumn by means of a transfer valve. The latter arrangement can be used for both sample cleanup and enrichment prior to chromatographic separation.
With regard to column packing, stationary phases can be classified according to the mechanism by which they separate molecules. Currently, the most popular type of column packing is the reversed (nonpolar) stationary phase on which analytes are retained and then eluted with a mobile phase or mobile phase gradient of sufficient polarity. Reversed phases have wide application and exhibit long lifetimes, good batch-to-batch reproducibility, low equilibration times, high mechanical stability, and predictable elution times and elution order. Ion-exchange stationary phases have limited application in food analysis, primarily for inorganic ions and for glyphosate.
Standard-bore and narrow-bore columns have internal diameters of ~4-5 mm and ~2 mm, respectively. Given the same stationary phase, a narrow-bore column can achieve the same resolving power as a standard-bore column in a shorter period of time and with less solvent, because the analytes can be eluted at a lower flow rate (<0.5 mL/min versus 2—3 mL/min) but at a comparable or faster linear rate. Moreover, narrow-bore columns can achieve solvent economies greater than 60% and are four to six times more sensitive when processing a standard- bore column sample injection volume. Narrow-bore columns place high demands on chromatographic equipment as precise, pulse-free flow is more difficult to achieve at lower flow rates. Dead volume must also be minimized throughout the system. Guard columns are recommended as the column frits are more likely to block.
INSTRUMENTATION AND DATA
When using HPLC in food applications, the selection of sample injection systems, pumps and detectors is just as important as it is with other demanding industrial analytical applications.
Sample Injection. Compared with manual injectors, automated sampling systems offer highly reproducible injection volume, low carryover and increased sample throughput. Modern autosamplers are designed for online sample preparation and derivatization, and in some cases, to safely handle highly corrosive solvents or mobile-phase additives. They may also offer cooling and heating capabilities to preserve unstable species or
to induce reactions.
Pumps. HPLC pumps must deliver pulse-free flow with high precision over a wide flow rate range with low dead volume. In addition, the pumping system must be able to precisely meter, mix and deliver a gradient mobile phase. Gradient pumps are either low-pressure (0.2 and 10 mL/min) or high-pressure (0.05 and 5 ml/mm). High-pressure gradient systems are able to form sharp gradient profiles and to change solvents rapidly (100% A to 100% B) without degassing. Typically, they comprise two isocratic pumps, each dedicated to one solvent. Low pressure gradient mixing is less expensive than the high-pressure alternative, but degassing is necessary for highest reproducibility.
Detectors. In food analysis, the limit of detection (LOD) and limit of quantification (LOQ), are defined, respectively, as two to three and 10 to 20 times the noise level. The LOD for food additives can be as low as 100 parts per trillion. Other important detection parameters are dynamic range, calibration linearity, chromatographic selectivity and qualitative information. A detector is considered selective if it does not respond to coeluting compounds that could interfere with analyte quantification. Table 2 outlines the relative characteristics of detectors commonly employed in food analysis.
HPLC analytes can often be identified on the basis of their retention times and either their UV-visible or mass spectra. Spectral information is especially useful in identifying coeluting peaks, which turn up fairly frequently in many food matrices. In such cases, diode array detection across multiple wavelengths or scanning across the mass spectrometry range enables deconvolution of overlapping peaks.
Derivatization may be required to improve sensitivity and/or selectivity of an optical detector (UV, visible or fluorescence) when the analytes lack chromophores or an adequate optical response. Precolumn is preferred to postcolumn derivatization because a separate reagent pump is not required and because reagents can be apportioned to each sample rather than being pumped continuously through the system. Derivatization can be automated and integrated online within the analysis.
Appropriate chromatography software can process data from several detectors simultaneously and generate both standard and customized reports that can be printed or stored electronically for inclusion in other documents. Database programs also are available to monitor quality control and chart trends in product quality.
CONCLUSION
Keeping track of the increasing diversity of food products with their ever-expanding profusion of additives is a daunting analytical challenge; one that must be continually met if we are to ensure the quality and safety of our food supply. HPLC is powerfully current because its fundamental principle—selection through differential molecular interaction—is based on fundamental variations in classes of properties across all chemical species. This selection principle continues to be a rich source of methodological innovation for analytical separation, detection and quantification.
Angelika Gratzfeld-Huesgen is an application chemist, liquid chromatography, with Agilent Technologies in Waldbronn, Germany. She can be reached at angelika_gratzfeld-huesgen @agilent.com.
Alan Schein is an Agilent Technologies science and technology writer/communications consultant focusing on analytical applications in several disciplines and industries.
The Application of HPLC in Food Analysis
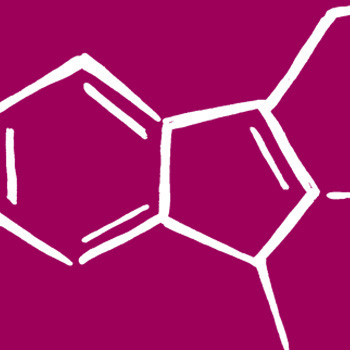