Bacteriocin-Producing Starter Cultures for Salami
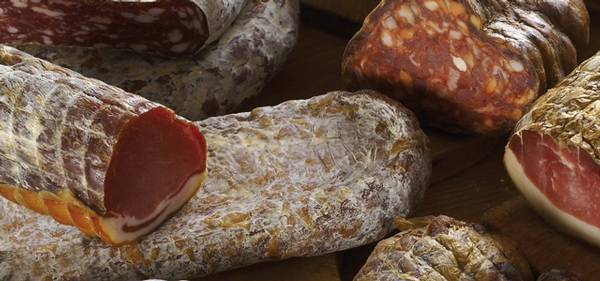
Microorganisms are a group of organisms that are both treasured and despised. They are well known and appreciated in, for example, production of dairy products and wine. They are detested because they cause a multitude of diseases, among them food poisoning, which leads to deaths every year. Furthermore, they cause huge economic losses due to reduced shelf life and spoilage of food products. Nevertheless, not many contemplate that human beings carry approximately 2 kg of microorganisms with more than 1014 CFU bacteria and a diversity of more than 1,000 species, ranging from harmless to pathogenic bacteria, in our gut. All these microorganisms are necessary and perform many important functions for our existence and well-being.
It is important to recognize that all not-sterile food contains microorganisms; nonetheless, only bacteria will be mentioned herein. The composition and level of bacteria are highly influenced by a variety of internal (e.g., bacteriological quality, food matrix, pH, water activity and fabrication) and external (e.g., humidity, packaging and temperature) features, in addition to synergistic and antagonistic interactions between these factors. For bacteria, the food matrix is important, as bacteria need nutrients and water to thrive, but the type of packaging, availability of oxygen and temperature during processing and storage highly affect both the composition and level of bacteria. Consequently, the bacterial biota is connected with the food product and storage conditions, and it is important to remember that the bacterial population is extremely diverse: what is bad for one might be good for another, as protection might target a limited group and, consequently, not be efficient. Even dormant bacteria might be of concern because, by either cross-contamination or a change of environment, they might reach a food matrix or conditions that allow activation and thereby trigger food poisoning.
For living bacteria, a certain level of pathogenic bacteria is needed to cause human food poisoning, but the amount required to cause illness depends highly on an individual’s physical condition. Here, there are interactions between, for instance, conditions of the immune system, use of medicines or alcohol, pregnancy and age. For already-known pathogenic bacteria, it is difficult to provide specific limits; nevertheless, guidelines are available. Not only might bacteria cause problems in food products, but also toxins produced by certain bacteria might be involved in food poisoning both before and after the pathogenic bacteria are eliminated. It appears to be a very complex and almost impossible task to contemplate bacteria in food products, and as mentioned, the presence of both spoilage and pathogenic bacteria results in huge economic losses due to discharged food and food poisoning. Luckily, the majority of bacteria are harmless, some even helpful, but why count on uncontrolled indigenous bacteria in food production when dominant and undamaging starter cultures might be an option?
Starter Cultures
Traditionally, lactic acid bacteria (LAB) have been used in the production of food, in dairy products as well as in meat products and vegetables. For early applications, LAB were characterized by their presence that resulted in changes in, for example, pH, texture and sensory characteristics of fermented food. More recently, LAB are applied as protective or probiotic cultures that don’t influence the sensory properties of the food products. LAB are generally recognized as safe (GRAS) organisms and well known for their ability to produce a number of antimicrobial compounds. None of these compounds need, by themselves, to elicit a complete inhibitory effect, but together or in the presence of other inhibiting factors, they can contribute to a hurdle effect. The understanding is that the sum of these protective factors results in the overall inhibiting effect. Protection of meat can be achieved by competitive exclusion, bacteriocin producers or a combination of both.
Meat Starter Cultures
Cured meat products, such as cured, fermented and dry sausages/salami and fresh sausages, are raw meat products; thus, there will always be an unknown amount and composition of indigenous bacteria in the meat matrix.
Salami was produced long before the outcome of microorganisms on the process of producing salami was recognized. Before starter cultures were commercialized, the manufacture of salami relied on the existence of indigenous LAB in raw materials. That was possible due to smaller, handmade “home productions.” However, it was difficult to transfer this production method to more industrial production. Problems such as long, variable production time, discoloration and off-flavor appeared.
As a way to circumvent these problems, meat manufacturers could presalt the meat to favor indigenous LAB to produce salami. Unfortunately, not all LAB are usable for this process. The “right” LAB are homofermentative, which produce lactic acid during fermentation. Unwanted LAB are heterofermentative, which also produce other organic acids and gas. Another way to overcome these problems is the usage of the back-slopping method (using a prefermented meat mince addition early in the processing). The limitation of this method is that the bacteria present in this meat mince might be unwanted ones, such as pathogenic Escherichia coli O157:H7 and E. coli O111, which then are reinoculated and may survive the manufacturing process. In addition, this method could favor the survival of indigenous antibiotic-resistant strains. Furthermore, if old pieces of dry sausage are added, the bacteria present may not be representative or very active as fermenting and ripening bacteria, and therefore neither way of processing is reliable.
Starter Cultures in Salami Processing
As a result, starter cultures were introduced about 40 years ago to the salami industry and are now widely used and accepted as a necessity to control processing and ensure quality in salami production. The advantages of meat starter cultures are wide-ranging: They lead to standardization and safety of the production process, achieving enhanced development of aroma, flavor and stable color, depending on the processing. It is possible to turn out product with a high degree of uniformity, and it might be possible to accelerate the processing time.
In the manufacturing of meat products, starter cultures are still primarily applied for technological reasons, such as adding LAB to enhance a controlled pH drop to ensure drying; nevertheless, they also are protective due to competitive exclusion, and they will inhibit some spoilage and pathogenic bacteria due to the drop in pH. The right combination of the recipe, starter culture and fermentation conditions makes it possible to control the composition and activity of the bacterial flora during the acidification and ripening of salami.
Another group of bacteria beneficial in the production of meat products is nontoxic staphylococci. They are applied to improve aroma formation, color development and color stability. Staphylococci will not only improve these features during the production of salami but likewise enhance their development in whole muscles, such as coppa, pancetta, bresaola and semidry ham products. The advantage of applying starter cultures in these categories of raw meat products is well established, as it is recognized that it is necessary—and advantageous—to control the indigenous biota with controlled and beneficial bacteria.
The activity of meat starter cultures is summarized in Table 1.
When it comes to safety, LAB are most advantageous; therefore, only LAB will be further discussed.
The antimicrobial properties of LAB consist of several activities that most likely interact and possibly have synergistic effects. Among the most powerful properties relevant for the production of salami are the following:
• Production of organic acids lowers pH; organic acids have an antimicrobial effect, as many spoilage and pathogenic bacteria are inhibited by a lowering in pH.
• Production of bacteriocins, heat-stable polypeptides produced by certain LAB, has an antimicrobial effect on other, mostly closely related bacterial species.
• Lowering the redox potential by the growth of LAB inhibits, in particular, indigenous, aerobic spoilage and pathogenic flora.
• Nutrient competition occurs when a high number of LAB are added. These bacteria quickly reduce the easily transformable nutrients, and consequently, indigenous bacteria are inhibited.
Bacteriocins
As mentioned, bacteriocins are antibacterial peptides, which may influence the growth of related bacteria in their near environment. Nearly 300 types of bacteriocins have been discovered, of which the ones produced by LAB are used commercially. The genes encoding bacteriocins are located on either chromosomes or plasmids, and those produced by LAB have a relatively broad spectrum of action. The bacteriocin-producing strains are protected against their own bacteriocin, as they possess genetically determined immunity genes.
There are different ways of classifying LAB bacteriocins; one of them is division into three main classes, with a number of subclasses. The classification is predominantly based on similarities in structure, mode of action and common characteristics. For food applications, class I and II bacteriocins are prevalent, and most research has been conducted on these bacteriocins.
Class I bacteriocins are called lantibiotics because they contain the amino acid lanthionine. They are small peptides synthesized as prepeptides and are divided into types A and B. Type A are elongated peptides, which inhibit or kill susceptible bacteria by forming pores via electrostatic interactions in membranes of target bacteria. Hence, the cell motive forces and equilibria are disrupted, and the bacteria inactivated. Nisin is the best-known class I type A bacteriocin. Type B are globular-shaped peptides that, by inhibiting enzymes in receptive bacteria, lead to destruction.
Class II bacteriocins are small, heat-stable bacteriocins with a high content of small amino acids; their mode of action is similar to class I type A bacteriocins with species-specific activity that causes cell leakage by permeabilizing the target cell wall. They are divided into four subclasses: a—d. Class IIa bacteriocins are produced by food-associated LAB isolated primarily from dairy and meat products. They have different antimicrobial activities but are commonly active against Listeria spp. Furthermore, they have the conserved amino acid sequence YGNGV and at least two cysteine residues that form S-S bridges, which seem to be vital for their activity. Pediocin PA-1A is a well-known class IIa bacteriocin. The main characteristic of class IIb bacteriocins is that they require two different peptides for activity. To this group belong various plantaricins and lactococcins.
Class III bacteriocins are large and heat labile; here, helveticin J may be mentioned.
As purified, commercially available bacteriocins, only nisin and pediocin are on the market. In purified form, they are considered food additives and therefore need regulatory approval. Within the EU today, only nisin, E234, is applicable to a limited range of food products, for example, types of cheese, puddings and canned food, but not to meat products.
Bacteriocin-Producing Bacterial Strains
As bacteriocins have a peptidic structure, the meat’s natural proteolytic system might inactivate them if added directly to raw meat products. Therefore, active metabolizing bacterial strains, which can produce bacteriocins during the fermentation process, are advantageous to achieve an inhibitory effect during the production of salami. Bacteriocin-producing salami cultures on the market consist of LAB strains, such as Pediococcus acidilactici, Lactobacillus sakei and Lactobacillus plantarum either alone or in combination, to achieve production of bacteriocin across a broad temperature range such that differences in their temperature growth optimums yield bacteriocin early and/or later in the fermentation process.
Generally, in properly fermented salami produced with a commercial starter culture, the built-in hurdles should control potential Listeria growth. Depending on processing, even a slight reduction in total Listeria might occur, but sufficient bacteria might survive to induce cross-contamination during further handling, such as slicing. The good news here is that Listeria monocytogenes is vulnerable to bacteriocins, and consequently, by applying a bacteriocin-producing starter culture, not only are the technological demands accomplished, but enhanced safety also is achieved. In addition to controlling acidification, which enhances drying out, thus providing a good texture, their protective properties enhance the control of Listeria and the production of bacteriocins ensures improved safety of salami produced with such starter cultures.
Listeria are very resistant to various physical and chemical influences, and consequently, are widely spread in nature and present in all types of raw materials. Generally, Listeria resist both high and low temperatures, and can resist freezing and drying. Furthermore, Listeria are very tolerant to pH and have also been known to aggregate as biofilms, being stubbornly resistant to antimicrobial agents, which consequently results in secondary contamination of food products. A risk factor in the production of food products is that up to 10% of the population are healthy carriers of L. monocytogenes, so without appropriate precautions, this organism might contaminate products during the production process.
Outbreaks of listeriosis from L. monocytogenes have been linked to cheese and raw materials as well as finished products manufactured from meat, poultry and fish. Symptoms can range from ailments similar to influenza to blood poisoning, meningitis and encephalitis. Furthermore, the pathogenic bacteria can cause abscesses or granulomas similar to those found in tuberculosis of internal organs. The incubation period can range from a few days up to several months. Afflictions occur particularly in people with weakened resistance and can therefore be fatal to people with compromised immune systems. Additionally, risk groups include the fetus, resulting in miscarriage, small children, pregnant women and the elderly. Mortality is more than 50% for people in these groups, whereas it is only a few percent for individuals with healthy immune systems. Therefore, although L. monocytogenes is widespread in the environment and serious infections are rare, death rates due to listeriosis are high with outbreaks.
Protective Starter Cultures
In recent times, considerable focus has been directed to the field of starter cultures for protection. “Protection with starter cultures” is the term used when the sole purpose of a culture is to suppress undesirable indigenous bacteria and thereby enhance the quality and safety of the meat products.
It has been found that most big food poisoning outbreaks were connected with “too bacteriologically clean” products, as without competitive and harmless flora, spoilage and pathogenic bacteria, such as Listeria, could develop rapidly. Protective cultures should also be competitive at low temperatures, because at this point, the indigenous flora will develop in cold-stored meat products. Furthermore, starter cultures should not considerably change the sensory properties of food products, but inhibit spoilage and pathogenic bacteria by competitive exclusion and/or bacteriocin production to increase safety and quality. This means that by adding a controlled LAB culture to applicable food products, it should be possible to suppress unwanted bacteria and thereby obtain a higher degree of product safety. However, it is not so easy to persuade manufacturers to apply “good” protective cultures, because the beneficial evidence is not technologically apparent or easily measurable. The starter culture concept is used mainly in fresh sausages, spreadable meat products and brine injected into whole muscles sold as fresh products. Protective cultures are also applied on the surfaces of processed meat products to minimize cross-contamination during handling. With suitable starter cultures, the potential for improved safety is extensive.
Many hurdles are traditionally used during the processing of meat products: salting, addition of nitrite or organic acids, cold smoking and packaging using either modified atmosphere or vacuum. Still, lightly preserved food products are high-risk products, especially when consumed without heat treatment, when it comes to the growth of unwanted bacteria, as the hurdles are not usually sufficient to control the indigenous flora present from the raw materials, processing and postprocessing/handling of the products. Nevertheless, it appears that most indigenous pathogenic bacteria in lightly preserved meat products may adequately be controlled by strictly respecting production hurdles, such as salt content, smoke and storage conditions. However, L. monocytogenes still remains a microbial risk. The prevalence is usually low initially, but the expiration date is often influenced by the risk of L. monocytogenes to exceed, for example, EU food safety criteria for ready-to-eat food within the tolerated limit of 100 CFU/g (EC 1441/2007).
Future Prospects
Commercially, the use of viable LAB as protective agents in food products is still viewed with skepticism, although researchers—obtaining positive results—have worked with this technology for a long time. Starter cultures with protective properties in, for example, salami production, should be unproblematic with regard to both application and legislation. However, in practice, the application of protective cultures for other meat applications, such as fresh and cooked meat products, is not simple. Among other things, the cultures should not be considered preservatives or as stand-alone additives, but rather as extra built-in safety factors within a hurdle system. Furthermore, for several products, it is a technical necessity to add an extra application step after cooking to utilize the culture, which could be an obstacle for some manufactures. It should also be mentioned that the application of protective cultures to meat products, such as bacon, smoked filet, emulsion products and other cooked meat products, is not part of general starter culture acceptance. This might be why supervisory authorities are not very understanding towards the high initial level of LAB in such products. Yet, there is strong scientific opinion that consumers will consider protective cultures a natural way to ensure the quality and safety of food products in the future.
Lone Andersen, M.Sc., works with Sacco S.r.l. in Italy and is responsible for building up their nondairy application field, including the use of starter cultures for production of fermented meat, vegetables, bread and silage and the use of probiotic bacteria in food applications.
Looking for a reprint of this article?
From high-res PDFs to custom plaques, order your copy today!