Indicator Organism Assays: Chaos, Confusion and Criteria
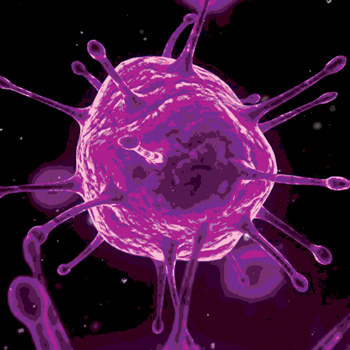
There is a great deal of confusion and unfortunate controversy about “indicator” organisms. For example, the term has been used to describe a (theoretical) non-pathogenic bacterial assay that, if negative in a sample, could be taken to mean the product is free of the indicated pathogen and, if present, indicates the possible presence of a pathogen. It has also been used as a measure of the hygienic or sanitary conditions of surfaces in a food processing environment.
Another usage is as an organism or test that reflects the microbiological “quality” of a product. It has also been used to describe an organism whose presence or level indicates the potential for future spoilage or as a “surrogate” organism, for example, those organisms that model the behavior of pathogens under certain conditions. In addition, some “indicators” are not specific organisms but assays for groups of organisms, such as coliforms, Enterobacteriaceae (EB), aerobic plate counts (APCs), etc. Is there any wonder that confusion and disagreement among food safety professionals abounds when the term “indicator” is used? Each of the aforementioned usages will be discussed in what this author believes to be their primary meaning, although there are specific circumstances where the terms may overlap.
Indicators of Pathogens (Index Organisms)
A means to ensure the microbiological safety of drinking water was needed at the turn of the 20th century. This approach had to ensure that water was free of fecal pathogenic bacteria, such as Salmonella. However, Salmonella assays were time-consuming and laborious at that time. It was understood that Escherichia coli was associated with the gastrointestinal tract of man and animals and that the E. coli assay was simpler to perform and more reliable at that time than the assay for Salmonella. It was believed that if water was contaminated with fecal matter that E. coli would be present and would persist longer than Salmonella in the water supply. Thus, E. coli became recognized as an “indicator” organism in place of the Salmonella assay. Additionally, E. coli became an “index” organism (the preferred term in this context1) for the possible presence of Salmonella or other pathogens of fecal origin. However, E. coli is a member of a methodologically defined group of organisms called “coliforms” (“coliform” is not a taxonomic term), and it was not long until water began to be tested for coliforms as well. This occurred and continues to occur today, even though most coliforms are not of fecal origin. In fact, E. coli is believed to be the only coliform of fecal origin, although this author has seen E. coli niches in several food processing environments that were not likely to have been directly contaminated with fecal matter (e.g., cooling coils on an overhead ceiling-mounted refrigeration unit in a cheese processing room). The term “fecal coliform” is misleading, especially as it relates to organisms other than E. coli. Gradually, this index organism/test concept was (wrongly) expanded to include other foods in which a microbe would not merely survive but could grow. The concept of an index organism (e.g., for a pathogen) has not withstood scientific inquiry (there is no perfect index organism/test), even with water samples over the past century.[1] Nevertheless, the concept persists and has some meaning in other contexts as described below. For example, there appears to be a correlation between index organism levels as measured by APCs and the presence of generic E. coli biotype I (an indicator of fecal contamination) on beef carcasses over multiple lots[2] and evidence that the same is likely true for pathogens (e.g., incidence of Verotoxin-producing strains of E. coli was correlated with log{10} APCs of bovine carcasses[3]). Kornacki and Johnson[1] presented data showing a correlation between levels of E. coli and incidence of Salmonella as an example of this sort of general correlation, although it is not a specific lot-to-lot correlation (Table 1). It should be noted that, although such index organism tests, where verified, are not reliable for individual production lots, they nevertheless point to potential pathogen issues when viewed over multiple lots. Thus, they have some utility if correctly interpreted. Their presence could be used to enhance existing food safety systems.
This concept provides some of the justification for the International Commission on Microbiological Specifications for Foods (ICMSF) sampling schemes as they relate to “indicator” assays (ICMSF Cases 4-6; Figure 1[4]) applied to foods as a measure of risk. The ICMSF sampling scheme is based upon risk associated with the product either from extrinsic (e.g., temperature, pressure treatments, handling, post-process contamination, etc.) or intrinsic factors (e.g., pH and water activity) vs. the risk associated with particular agents (e.g., spoilage, indicator, moderate, serious and severe organisms or toxins, etc.).
Indicators of Hygiene and Sanitation
Very often, the term “indicator” is used to describe a test or organism used to measure the hygienic conditions of surfaces in a food production facility before, during and after operations (see Table 2). However, different specifications would apply to these conditions. For example, a pre-operational (post-sanitization) surface should be free of coliforms, EB, yeast and molds, since these organisms are very sensitive to the action of sanitizers. Hence, if they are discovered, investigation as to the effectiveness of soil removal that often entraps and protects microbes should be undertaken. However, no facility can be expected to be maintained under sterile conditions. Consequently, some APCs, psychrotrophic plate counts and mesophilic spore counts taken on pre-operational surfaces can be expected. Good targets for large (e.g., 1 ft[2]) pre-operational surfaces are less than 100 or less than 1,000 APCs with less than 10 coliforms or EB and less than 10 yeast and molds. However, some use different values for different surface areas (e.g., 4×50 cm[2]). Evancho et al.[5], quoting the U.S. Public Health Service as providing a guide of less than 100 colonies recovered per utensil or surface area of equipment sampled, added that the interpretation from unmeasured surfaces (e.g., utensils, gaskets, pump impellors, etc.) should be based upon historical data gained from these when sampled after documented thorough cleaning and sanitization.
Operational and post-operational surfaces can be expected to have higher hygienic indicator counts, particularly on surfaces that have been in contact with moist product residues. However, it is unlikely that sites initially with less than 1,000 colony-forming units (CFUs) per ft[2] will become contaminated with 100,000 or more CFUs by incidental means (e.g., air or contact with clean hands, etc.). Such an increase in population is the result of inadequately controlled microbial growth.[4]
Truncated pathogen assays as hygienic indicators
These assays are characterized by truncating or modifying a standard pathogen assay to include broader microbial groups that may contain the pathogen of concern but also indices of hygiene and product quality. These positive assays are not “presumptive positive” pathogen assays. The best known example of this is the test for Listeria-like organisms, wherein the standard assay is stopped at the Fraser broth or Modified Oxford medium (MOX) plate steps as in Figures 2 (secondary enrichment and selective medium steps, respectively), 3 and 4. “Listeria-like” microbes, for example, those that hydrolyze esculin in Fraser broth (as shown by blackened tubes) or typical black colonies on MOX agar, could include Listeria spp., Enterococcus spp., Lactobacillus spp., among others.[6,7] The finding of organisms within the broad group of “esculin-hydrolyzing bacteria” (i.e., “Listeria-like organisms”) on a pre-operational surface indicates that improvements in cleaning and sanitation are needed, but they do not directly indicate that the surface is contaminated with a pathogen. Should a pre-operational surface test negative, such data would provide evidence that the surface is free of selected Gram-positive microbes, including Listeria monocytogenes. Should such a surface test positive, all implicated product lots should be tested as appropriate for Listeria in a statistically relevant manner in accordance with the appropriate U.S. Food and Drug Administration (FDA) or U.S. Department of Agriculture (USDA) guidance, and appropriate actions taken, depending on the result. The presence of L. monocytogenes would indicate an adulterated lot.
Baird-Parker agar
Frank et al.[8] showed that 85% of 47 Listeria spp.-positive environmental samples among 409 samples tested from 15 dairy plants recovered more than 6,600 lecithinase-positive, typical black colonies per sampled area from Baird-Parker agar. Kornacki[9,10] showed that a number of strains of Listeria spp. also appear as ‘typical’ black colonies on Baird-Parker agar without a zone of precipitate. Baird-Parker agar is non-specific and can pick up colonies of Microcococcus spp., Staphylococcus spp., Listeria spp., a strain of Pediococcus (since renamed as an Enterococcus spp.[9,10]) and even Bacillus spp. (although they look very atypical on Baird-Parker agar). Consequently, Baird-Parker agar could be a valuable tool on pre-operational sampling of Zone 1 and 2 surfaces. This quantitative approach has the advantage of recovering a broad spectrum of organisms, but lacks sensitivity that comes from an enrichment-based approach.
Hydrogen sulfide-producing strains of EB
The Grocery Manufacturers Association has stated, “A suitable indicator for Salmonella has not been identified.”[11] A truncated Salmonella assay would not be appropriate in this instance since it would yield a “presumptive positive” Salmonella isolate according to some interpretations of the method. Some use an EB count as a hygienic indicator, but there is no correlation between EB count and Salmonella.[1] The EB count is too broad (includes too many genera) and too insensitive because it is only a quantitative but not qualitative (enrichment-based) assay. Traditional Salmonella assays are qualitative; hence, their sensitivity is greater. Recently, Kornacki[12] described an approach for the detection of microorganisms of similar physiology to Salmonella that is an enrichment (thus overcoming the sensitivity issue) but is more restrictive to those EB that are physiologically similar to Salmonella.
Indicators of Quality, Cross-contamination and Process Control
Ready-to-eat foods that have undergone a biocidal Critical Control Point (CCP) (e.g., smoke house treatment with meats, pasteurization of fluid dairy products, etc.) should not contain organisms that are destroyed by those treatments unless the process failed or the product has been contaminated from the environment (post-process contamination). Consequently, the presence of coliforms, yeast and mold counts and EB in product from an appropriately validated smoke house or appropriately pasteurized milk would be an indicator of post-process contamination. Their presence alone does not indicate that the product is dangerous but could reflect room for improvements in the processing environment to reduce post-process contamination resulting from operating, maintenance/repair or equipment/facility design practices.[4] Appropriate guidelines for quality or cross-contamination indicator organism testing of foodservice items are available.[13]
This author recalls a situation wherein generic E. coli was consistently recovered from an oven-cooked meat product. EB populations in raw product going into the oven averaged about 200 per gram. Samples were selected at the exit of the oven, and 375-gram quantities cooled immediately and enriched with nine parts of lactose broth each. Upon incubation and streaking, three of 10 samples tested positive for the presence of EB, clearly indicating a process failure, despite mean surface product temperatures suggesting otherwise. Further investigation revealed portions of the product were undercooked, only achieving about 113 °F, and did not reach the mean surface temperature recorded. In this instance, the EB count was used as a means to monitor the efficacy of the process in a particular context.
Indicators of Spoilage
Not all food spoilage is a consequence of microbial activity. However, a number of microorganisms cause spoilage in a variety of ways. Spoilage indicators could be considered a subset of quality indicators as microbes that cause spoilage are not necessarily those that result in foodborne illness. A review of spoilage defects and the microbes that cause them is available.[14] There is still a need in many parts of the food industry to understand the speed at which a product will spoil despite a good deal of research on predictive microbiological spoilage. This is a difficult problem that is influenced by a multiplicity of factors, for example, microflora in the processing facility (the same process in a different place may have different microflora), the levels and frequency of post-process contamination, ingredient quality, product storage time and temperature, product matrix, pH, humidity, growth rate and physiology of the spoilage microbe, etc. In many instances, a normally refrigerated product is incubated at an elevated temperature to accelerate the growth of spoilage organisms, and then an assay is performed. This is the principle behind the Moseley test and the preliminary incubation assay for milk.[15] However, there is a limit to how high a temperature one can incubate the sample, as many spoilage microbes are psychrotrophic and have optimum growth temperatures lower than that of typical mesophilic facultative anaerobes. While other predictive models for spoilage have been developed for other products,[16] they are beyond the scope of this article. However, it is this author’s conviction that determinations of product shelf life should be based upon studies with product manufactured in the plant and not a pilot plant where the contaminating microflora may be different or better controlled.
Surrogate Microorganisms
Surrogate organisms “are harmless microbes with correlated survival and growth parameters to specific patho-gens.”[4] They are frequently used to validate the efficacy of Critical Limits for CCPs, can be naturally occurring or artificially added and should be non-pathogenic. Both the USDA and FDA have acknowledged the use of surrogate microorganisms as a valid approach where appropriate.[4]
Naturally occurring surrogates (an example)
If one has a robust thermal process and a high population of naturally occurring, verified, heat-resistant organisms, then a demonstrated five-log{10} reduction in the product matrix under minimal conditions of operation should be adequate to destroy at least that many vegetative pathogens of lesser heat resistance. However, it is critical that one has validated in a laboratory that the naturally occurring surrogates are more heat resistant than the pathogens of concern in the product under the temperatures and times consistent with the process. Examples of this type of approach have been previously described.[4]
Artificially inoculated surrogates
Research on the development and appropriate use of artificially inoculated surrogates is still needed. Thermal surrogates have been characterized for some foods under certain conditions.[17-19] However, validation that the surrogate is appropriate (e.g., equivalent, greater or correlated heat resistance to the pathogen of concern) in the product matrix should be done before studies are undertaken under plant or pilot plant processing conditions. Despite the name, Pediococcus sp. NRRL B-2354 (Enterococcus sp. NRRL B-2354) “is a nonpathogenic spoilage organism that has been used as a test organism in milk pasteurization studies.” The authors go on to state that “These characteristics make this bacterium an attractive test organism to study the mode of bacterial thermal inactivation in a food pilot plant.”[20]
In Conclusion
The phrase “indicator organisms” really encompasses multiple concepts and is often left undefined in conversation. It is a concept about which there are many opinions and opportunities for misunderstanding and misapplication. The term “indicator organisms” has been used to mean “index” organisms, indicators of hygiene and sanitation on equipment and environmental surfaces, of product quality, of process control and of spoilage or spoilage potential and also as surrogates in the context of CCP validation. There may be circumstances where more than one meaning may be applicable. It is hoped that this article, rather than promoting further confusion, will serve to provide a basis for discussing this important topic.
Jeffrey L. Kornacki, Ph.D., is president of Kornacki Microbiology Solutions, Inc. in McFarland (Madison), WI. Dr. Kornacki has performed in-factory microbiological investigations, risk assessments and food safety-related audits for 21 years in over 500 food-processing facilities in a variety of capacities with different organizations. Between 2001 and 2003, he was an assistant professor at the University of Georgia’s Department of Food Science and Center for Food Safety prior to starting several food safety consulting companies and remains an adjunct assistant professor in the University of Georgia’s Department of Food Science. He has researched, published and spoken widely on pathogen control, testing and sampling in food production facilities.
References
1. Kornacki, J. L. and J. Johnson. 2001. Enterobacteriaceae, Coliforms, and Escherichia coli as Quality and Safety Indicators, Chapter 8. In: Downes, F. P. and K. Ito (eds) Compendium of Methods for the Microbiological Examination of Foods, 4th Ed. American Public Health Association, Washington, D.C. pp. 69-82.
2. Siragusa, G., W. J. Dorsa, C. N. Cutter, G. L. Bennett, J. E. Keen and M. Koohmaraie. 1998. The Incidence of Escherichia coli on Beef Carcasses and Its Association with Aerobic Mesophilic Plate Count Categories during the Slaughter Process. J Food Prot 61:1269-1274.
3. Siragusa, G. R. 2001. Relationship between Microbial and Non-microbial Indicators of Fecal Contamination. International Association for Food Protection Annual Meeting, Minneapolis, MN. August.
4. Kornacki, J. L. 2010 (ed). Principles of Microbiological Troubleshooting in the Industrial Food Processing Environment. Springer, New York.
5. Evancho, G. M., W. H. Sveum, L. J. Moberg and J. F. Frank. 2001. Microbiological Monitoring of the Food Processing Environment, Chapter 3. In: Downes, F. P. and K. Ito (eds) Compendium of Methods for the Microbiological Examination of Foods, 4th Ed. American Public Health Association, Washington, D.C.
6. Kornacki, J. L., D. J. Evanson, W. Reid, K. Rowe and R. S. Flowers. 1993. Evaluation of the USDA Protocol of Detection of Listeria monocytogenes. J Food Prot 56:441-443.
7. Yan, Z., J. B. Gurtler and J. L. Kornacki. 2006. A Solid Agar Overlay Method for Recovery of Heat-Injured Listeria monocytogenes. J Food Prot 69:428-431.
8. Frank, J. F., R. A. N. Gillett and G. O. Ware. 1990. Association of Listeria spp. Contamination in the Dairy Processing Plant Environment with the Presence of Staphylococci. J Food Prot 53:928-932.
9. Kornacki, J. L., J. B. Gurtler, Z. Yan and C. M. Cooper. 2003. Evaluation of Several Modifications of an Ecometric Technique for Assessment of Media Performance. Abstract T01. International Association for Food Protection Annual Meeting, August 10-13. New Orleans.
10. Kornacki, J. L., J. B. Gurtler, Z. Yan and C. Cooper. 2003. Evaluations of Several Modifications of an Ecometric Technique for Assessment of Media Performance. J Food Prot 66:1727-1732.
11. Grocery Manufacturers Association. 2009. Control of Salmonella in Low Moisture Foods. February 4 (minor revisions March 16).
12. Kornacki, J. L. 2010. International Association for Food Protection Symposium S22. Food and Food Environment Test Considerations in View of Changing Regulations. (Organizer and Co-convener). Presentation entitled, “An Indicator Approach to Enteric Contamination of at Risk Foods.” August 1-4. Anaheim, CA.
13. Kornacki, J. L. 2011. Practical Sampling Plans, Indicator Microorganisms, and Interpretation of Test Results from Troubleshooting, Chapter 25. In: Hoorfar, J. (ed), Rapid Detection, Characterization and Enumeration of Foodborne Pathogens. ASM Press, Herndon, VA. (manuscript in press).
14. Clavero, R. 2010. Solving microbial spoilage problems in processed foods, Chapter 3. In: J. L. Kornacki (ed) Principles of Microbiological Troubleshooting in the Industrial Food Processing Environment. Springer, New York. pp. 63-78.
15. Duncan, S. E., B. R. Yaun and S. S. Sumner. 2004. Microbiological Methods for Dairy Products, Chapter 9. In: Wehr, H. M and J. F. Frank (eds) Standard Methods for the Examination of Dairy Products, 17th Ed.
16. Koutsuomanis, K. 2001. Predictive Modeling of the Shelf Life of Fish under Nonisothermal Conditions. Appl Environ Microbiol 67:1821-1829.
17. Ma, L., J. L. Kornacki, G. Zhang, C.-M., Lin and M. P. Doyle. 2007. Development of Thermal Surrogate Microorganisms in Ground Beef for In-plant Critical Control Point Validation Studies. J Food Prot 70:952-957.
18. Kornacki, J. L. 2006. International Association for Food Protection Symposium S07. Surrogate Microorganisms: Selection, Use and Validation (Organizer and Co-convener). Presentation entitled, “Examples of Surrogate .Validation and Use in the Industry.” August 14, Calgary, CAN.
19. Almond Board of California. 2007. Guidelines for Process Validation Using Enterococcus faecium NRRL B-2354. October 23. Surrogate Validation Guidelines v1.2.
20. Annous, B. A. and M. F. Kozempel. 1998. Influence of Growth Medium on Thermal Resistance of Pediococcus sp. NRRL B-2354 (formerly Micrococcus freudenreichii) in Liquid Foods. J Food Prot 61:578-581.
Looking for a reprint of this article?
From high-res PDFs to custom plaques, order your copy today!