The first report concerning Campylobacter was written in 1886 by Theodor Escherich, describing nonculturable, spiral-shaped bacteria. Later in 1927, a group of bacteria isolated from the feces of cattle with diarrhea was classified as Vibrio jejuni, and in 1944, Vibrio coli was classified when isolated from the feces of a pig with diarrhea. However, due to its microaerophilic growth requirements and nonfermentative metabolism, in 1963, the genus Campylobacter was for the first time proposed as distinct from Vibrio spp. Later, concerns over Campylobacter were raised by the study of Butzler et al. in 1973,[1] which described a high occurrence of the bacteria in human diarrhea.
Today, Campylobacter has been found to be the most common bacteria causing human gastroenteritis in the world. Many countries have invested heavily in and have been working over the past 10 years on reducing the level of contamination with Campylobacter, with some success. Despite these efforts, Campylobacter contamination remains at the top of all foodborne illnesses in humans and is still difficult to prevent.
According to the UK Food Standards Agency (FSA), Campylobacter food poisoning is the most prevalent foodborne illness in the UK, with an estimate of over half a million cases per year causing gastroenteritis, also known as campylobacteriosis.[2] Out of that estimation, 72,000 cases have been confirmed by laboratory analysis, and approximately 100 people have died.[3]
Campylobacter
Campylobacter species are paradoxical organisms; they are unable to multiply in food, in conditions below 30 °C, in air because of high oxygen levels or in dry conditions. So why are these organisms such a problem? Campylobacter has a widespread distribution and is most commonly found in poultry and wild birds but is also found in domesticated and wild animals, unpasteurized milk, untreated waters and shellfish.[4] However, the most common hosts with the highest level of Campylobacter spp. are birds, due to their high body temperature. Freezing poultry, at –15 °C and lower, can reduce the levels of Campylobacter spp., inactivating them in as few as 3 days.[5] But there is an issue of customers’ acceptance of frozen poultry in the UK market.
Campylobacteriosis usually develops a few days after eating food contaminated with Campylobacter spp. and leads to symptoms that include abdominal pain, severe diarrhea and sometimes vomiting. The duration of the illness after the incubation period and initiation of symptoms is 2 days and can be up to 2 weeks. It can affect some people permanently, causing irritable bowel syndrome, reactive arthritis and, in rare cases, Guillain-Barré syndrome—a serious condition of the nervous system—or Miller-Fisher syndrome, a chronic form of paralysis that, in the worst cases, can even result in death.[6]
In general, the treatment of patients infected with Campylobacter is to replace lost fluids and electrolytes. However, for more severe cases, antibiotics are used. There is a concern with Campylobacter in regard to the increase of antimicrobial resistance in various regions around the world. High levels of resistance to fluoroquinolones have been attributed to the use of this drug to treat farmed poultry.[7]
Research published in 2015 from 254 samples found that half of the Campylobacter strains tested proved resistant to at least two classes of antimicrobials (tetracyclines and quinolones).[5] This obviously poses a high risk for public health, because infection with antimicrobial-resistant Campylobacter may lead to suboptimal outcomes of antimicrobial treatments or even treatment failure in immunocompromised people.[8]
Slaughterhouses
Source-attribution studies, outbreak investigations and case-control reports all incriminate poultry as a major source of the foodborne transmission of Campylobacter infection. Transmission to humans is most often associated with the handling and consumption of poultry that has been contaminated during slaughter and carcass processing in slaughterhouses.[8]
A study in 2015 revealed the ease with which poultry carcasses are contaminated during slaughter from either mishandling or cross-contamination. It was also claimed that Campylobacter inevitably finds a way to the surface of the chicken meat when carcasses are contaminated with intestinal contents during plucking and evisceration.[5]
The method used to slaughter poultry has become a complex, rapid and highly automated process, which poses a major challenge with regard to contamination with Campylobacter spp. during slaughter.[9] Therefore, it is crucial during meat production to adhere to strict practices of slaughter hygiene, as well as risk-based preventive measures, to ensure high meat quality and protect public health. Research has shown that if all broiler batches from slaughterhouses complied with the maximum limit of 1,000 CFU/g, the risk to public health could be reduced by more than 50 percent.[4]
FSA Guidelines and Targets
Although Campylobacter species do not normally grow in food, these bacteria spread easily and have a low infective dose, between 53 and 750 CFU/cm2 in fresh chicken meat, meaning that only a few bacteria in a piece of undercooked chicken, or bacteria transferred from raw chicken onto other ready-to-eat foods, can cause illness.[10]
Because the risk to consumers appears to be associated with exposure to the number of Campylobacter microorganisms in food, FSA[2] has suggested the following categories for Campylobacter counts in chicken, which are restricted to only three levels for simplicity, allowing easy interpretation of results when monitoring against the baseline:[3]
• > 1,000 CFU/g
• 100–1,000 CFU/g
• < 100 CFU/g
Following this classification, FSA set a target in the UK to reduce the percentage of slaughterhouse poultry contaminated with more than 1,000 CFU/g of Campylobacter from a baseline of 27 percent in 2008 to 10 percent by December 2015.[4] Furthermore, FSA has initiated a program dealing with the whole food supply chain, from farm to fork, known as the “Acting on Campylobacter Together” campaign to reduce the spread of Campylobacter spp.
Test Procedures Carried Out to Detect the Current Level of Campylobacter
A total of 27 whole chickens and 25 packets of chicken liver were purchased from eight different locations in North London: four supermarkets (locations 1–4) and four local meat shops (locations 5–8); three to five samples were taken from each location. There was no chicken liver available from location 8 because they were not selling it.
On the day of purchase, two 10-g portions from each whole chicken sample were aseptically removed, one from the neck skin and one from the back skin (near the leg) as well as a 10-g portion from each chicken liver packet.
Previous studies have analyzed samples from the neck skin of the chicken. However, in this study, samples from the back of the chicken were also included. Because evisceration of the birds is done through the back, there is thus the possibility of a higher level of contamination in that region.
Next, the samples were mixed with 90 mL of Maximum Recovery Diluent and homogenized under aseptic conditions. A further dilution (10-2) was also prepared. Both dilutions were spread onto chromogen-like agar plates and incubated at 41 °C for 48 hours in a microaerophilic environment using a gas-generating kit specific for Campylobacter.
The first testing method was a recently developed chromogen-like agar, as described by Ahmed et al.,[10] which was used for the enumeration of Campylobacter in those samples. It is a novel agar medium that has been specifically designed for easy enumeration of Campylobacter coli and Campylobacter jejuni from poultry. However, this particular agar does not contain a chromogen; instead, it contains and relies on a nonspecific indicator called tetrazolium red. This dye reacts with the colonies’ growth and is converted into a formazan compound, which makes the colonies turn dark red on the clear agar, thereby making the identification and counting of these two particular Campylobacter strains found in poultry significantly easier.
Other test methods were performed to confirm the identity of the colonies. The isolated samples from the agar plates were examined for their motility as well as Gram stain reaction. The oxidase test was also carried out, but to avoid the interference of the tetrazolium red with the oxidase test reagents, the colonies from the chromogen-like agar were first streaked on horse blood agar plates and incubated microaerobically for 24 hours before testing for oxidase.
Lastly, to confirm the genus and species, primers for Campylobacter and C. jejuni were used in a polymerase chain reaction (PCR) to identify the isolates. Genus-specific PCR was performed using the method described by Linton et al.,[11] and the species-specific identity of C. jejuni was confirmed using the method described by Wang et al.[12] DNA amplification for both was carried out in a Biometra T3000 thermocycler using the method of Wang et al.[12]
Prevalence of Campylobacter Contamination
The FSA food chain analysis project concluded that the greatest risk of Campylobacter infection for people extended from the primary production to the retail sales of poultry meat and suggested various methods to reduce such hazards.
From the current research, the enumeration results from the plating method were converted into log10 CFU/g and are presented in Figure 1.
At first glance, it may seem that between the supermarkets (locations 1–4) and the local meat shops (locations 5–8), there were no significant differences. However, looking closely, the local meat shops had higher average counts (6,918 CFU/g) than the supermarkets (741 CFU/g), a massive difference. Furthermore, a similar trend was noticed with the liver samples. The average liver counts at the meat shops were 1,349 CFU/g and 525 CFU/g for the supermarkets. The percentages of these samples that fall within the FSA categories are shown in Table 1[2].
Such differences could be attributed to more hygienic slaughter for supermarket chickens and adoption of improved systems that reduce counts after evisceration. A reduction of 2 logs in chickens results in a significant decrease in the number of human cases of foodborne illness.[3]
Research by Bolton13 showed that Campylobacter spp. are particularly sensitive to desiccation and reduced on pork, lamb and beef at slaughter because the carcasses are air-chilled, which dries the surface. For poultry slaughter, the carcasses are water-chilled, maintaining a wet surface and facilitating the survival of Campylobacter spp.
Seliwiorstow et al.[8] showed that when one slaughterhouse used just water-immersion chilling, Campylobacter contamination was not reduced. However, when air chilling was used instead, a significant reduction in Campylobacter counts was demonstrated, suggesting that the duration of air chilling is an important factor.
Subsequent to a rigorous scientific evaluation, FSA described an alternative control measure that reduces the level of contamination by 2 logs at the slaughterhouse level, showing that spraying the carcasses with a mixture of acidified sodium chlorite and citric acid could lead to an overall reduction of 2.1 logs.[2]
Other recent research reports have concluded that there is a relation between the operational hygienic performance of poultry slaughterhouses and the contamination level of Campylobacter on poultry carcasses. Therefore, enhancing slaughter operations can reduce contamination. This finding signifies that it is crucial to adhere to strict practices of slaughter hygiene to ensure public health protection during meat production.[9]
Consistent with reported cases by the European Food Safety Authority[6] in the EU, 80.6 percent of the isolates were confirmed to be C. jejuni, and 7.1 percent were C. coli. For that reason, the novel agar used in this research was found to be the most suitable, as it is selective with a high recovery rate for these two strains. However, traditional microbiological methods for the detection and quantification of Campylobacter based on selective culture can be time-consuming and will not detect microorganisms in a viable but nonculturable state that might be infectious. While the use of newer and faster technologies such as PCR seems promising for the accurate detection and quantification of microorganisms, it must be noted that quantification of Campylobacter by PCR analysis can be very difficult without enrichment.
A selection of three isolates from chicken neck samples with the highest levels of contamination was examined to confirm the genus and species. All isolates produced sharp bands for Campylobacter genus-specific primers (Figure 2, left), and two out of three were amplified with species-specific primers for C. jejuni (Figure 2, right). The identity of the third sample (location 5, lane 5 in Figure 2, right) was not confirmed at the species level.
Impact of Interventions
The use of chlorinated water for processing carcasses has reduced numbers of Campylobacter on those carcasses. However, the use of chlorine in concentrations higher than those used in potable water is not permitted in the EU. FSA guidelines suggest a strict risk management control at the farm and slaughterhouse levels, built on Good Hygiene Practices (GHPs) and control of hazards.[14]
There is also cross-contamination between each flock that is processed at the slaughterhouse; to reduce Campylobacter contamination in poultry by infected broiler flocks, logistical measures must be implemented, and slaughtering of Campylobacter-positive flocks must be done after slaughter of Campylobacter-negative flocks. For this to be possible, a rapid Campylobacter detection method is required. Current culture-based detection for Campylobacter takes at least 2 days. Several alternatives have been developed, with the most preferred being real-time PCR detection methods.[15]
Additionally, knowledge about Campylobacter antimicrobial resistance at different levels of poultry production is important for the development of effective control strategies. Several studies have been conducted to investigate the prevalence of anti-Campylobacter susceptibility patterns, with most reporting the emergence of resistant strains, especially to quinolones. Therefore, the use of antibiotics at the farm level must be reconsidered, proving that slaughterhouses are the most critical control point between farming of poultry and consumers.
Summary
In conclusion, based on the small number of samples examined in this study, the level of contamination with Campylobacter spp. is very high in chicken and chicken liver samples in the UK, with a significant amount at the highest level set by FSA.
FSA reported a 27 percent prevalence in the highest category (> 1,000 CFU/g) and had set a target to reduce it to 10 percent by December 2015. However, our results indicate that 44 percent of chicken neck samples and 52 percent of chicken liver samples are still at that highest level.
Furthermore, our results suggest that local meat shops are more likely to sell products with higher levels of contamination compared with supermarkets. Nevertheless, the presence of Campylobacter is risky due to its low infective dose, which emphasizes the urgent need to avoid cross-contamination before and throughout cooking.
However, public safety should not rely upon cooking only. Farms and slaughtering processes must implement strict GHPs as well as the careful use of antibiotics.
Additionally, retailers should have checks in place to ensure that they are selling a safe product coming from slaughterhouses. More inspection controls should be put in place by local government for local butcheries and meat shops to bring levels down as well.
Gheorghe Nicorici, M.Sc., obtained an M.Sc. in food science from London Metropolitan University and a B.A. (Honors) in international culinary arts. In 2014, he became a member of the Institute of Food Science and Technology. He is currently looking to begin a Ph.D. program in food science and continue with Campylobacter research.
Hamid B. Ghoddusi, Ph.D., is a senior lecturer and researcher in food microbiology, biotechnology and health, and is currently the head of the Microbiology Research Unit and food science course leader at London Metropolitan University. His research interests are probiotics and prebiotics, food safety and traditionally fermented foods.
References
1. Butzler, JP et al. 1973. “Related Vibrio in Stools.” J Pediatr 82(3):493–495.
2. www.food.gov.uk/sites/defaμlt/files/multimedia/pdfs/campyloconf.pdf.
3. www.food.gov.uk/sites/defaμlt/files/multimedia/pdfs/campytarget.pdf.
4. www.food.gov.uk/news-updates/news/2015/14003/campylobacter-survey-results-12months.
5. Wieczorek, K, E Denis and J Osek. 2015. “Comparative Analysis of Antimicrobial Resistance and Genetic Diversity of Campylobacter from Broilers Slaughtered in Poland.” Int J Food Microbiol 210:24–32.
6. European Food Safety Authority. 2011. “EFSA Panel on Biological Hazards (BIOHAZ) Scientific Opinion on Campylobacter in Broiler Meat Production: Control Options and Performance Objectives and/or Targets at Different Stages of the Food Chain.” EFSA J 9(4).
7. apps.who.int/iris/bitstream/10665/80751/1/9789241564601_eng.pdf.
8. Seliwiorstow, T, J Baré, I Van Damme, M Uyttendaele and L De Zutter. 2015. “Campylobacter Carcass Contamination Throughout the Slaughter Process of Campylobacter-Positive Broiler Batches.” Int J Food Microbiol 194:25–31.
9. Zweifel, C, D Althaus and R Stephan. 2015. “Effects of Slaughter Operations on the Microbiological Contamination of Broiler Carcasses in Three Abattoirs.” Food Control 51:37–42.
10. Ahmed, R, CG Léon-Velarde and JA Odumeru. 2012. “Evaluation of Novel Agars for the Enumeration of Campylobacter spp. in Poultry Retail Samples.” J Microbiol Methods 88:304–310.
11. Linton, D, RJ Owen and J Stanley. 1996. “Rapid Identification by PCR of the Genus Campylobacter and of Five Campylobacter Species Enteropathogenic for Man and Animals.” Res Microbiol 147:707–718.
12. Wang, G, CG Clark, TM Taylor, C Pucknell, C Barton, L Price, DL Woodward and FG Rodgers. 2002. “Colony Multiplex PCR Assay for Identification and Differentiation of Campylobacter jejuni, C. coli, C. lari, C. upsaliensis, and C. fetus subsp. fetus.” J Clin Microbiol 40:4744–4747.
13. Bolton, DJ. 2015. “Campylobacter Virulence and Survival Factors.” Food Microbiol 48:99–108.
14. Allen, VM, SA Bull, JEL Corry, G Domingue, F Jørgensen, JA Frost, R Whyte, A Gonzalez, N Elviss and TJ Humphrey. 2007. “Campylobacter spp. Contamination of Chicken Carcasses during Processing in Relation to Flock Colonization.” Int J Food Microbiol 113:54–61.
15. de Boer, P, H Rahaoui, RJ Leer, RC Montijn and JMBM van der Vossen. 2015. “Real-Time PCR Detection of Campylobacter spp.: A Comparison to Classic Culturing and Enrichment.” Food Microbiol 51:96–100.
Prevalence of Campylobacter Contamination in Raw Chicken and Chicken Liver at Retail
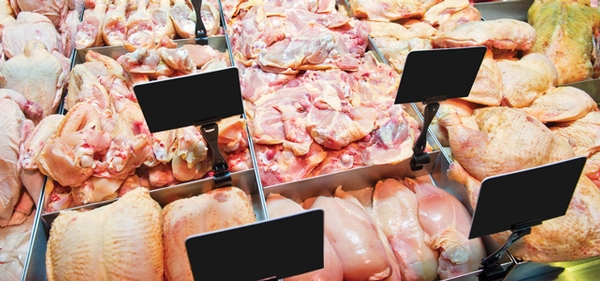
Looking for a reprint of this article?
From high-res PDFs to custom plaques, order your copy today!